The social mindset is the result of a massive duplication of a protein domain known as the Olduvai domain that occurred in ancestral human lineages over a relatively short period of time, beginning around 3.5 million years ago and reaching modern levels (around 270–300 copies) by around 1 million years ago.
The Olduvai domain is found in a family of centrosomal genes known as the NBPF family, whose genes were also subject to duplication.
There are six subtypes of the Olduvai domain. Out of these six, the three linked to the human increase in the social mindset have been termed HLS subtypes, for ‘human-lineage-specific (increases)’. The other three subtypes (CON subtypes, for ‘conserved‘), especially CON1, also experienced less numerous duplications.
Additionally, in one study, the effect on brain size was found to be strongest per copy for the less numerous CON subtypes, especially CON1. In humans, higher CON1 copy number in comparison to HLS copy number has been associated with lower social capacity, which is discussed on Genetics of our lack of the social mindset.
Introduction – microtubule-related proteins
Microtubules
Microtubules are cytoskeletal structures made of tubulin proteins and are responsible for physically carrying out the process of cell division. They are also a significant agent in determining cell shape[1][2] and in the migration of most cells.[3]
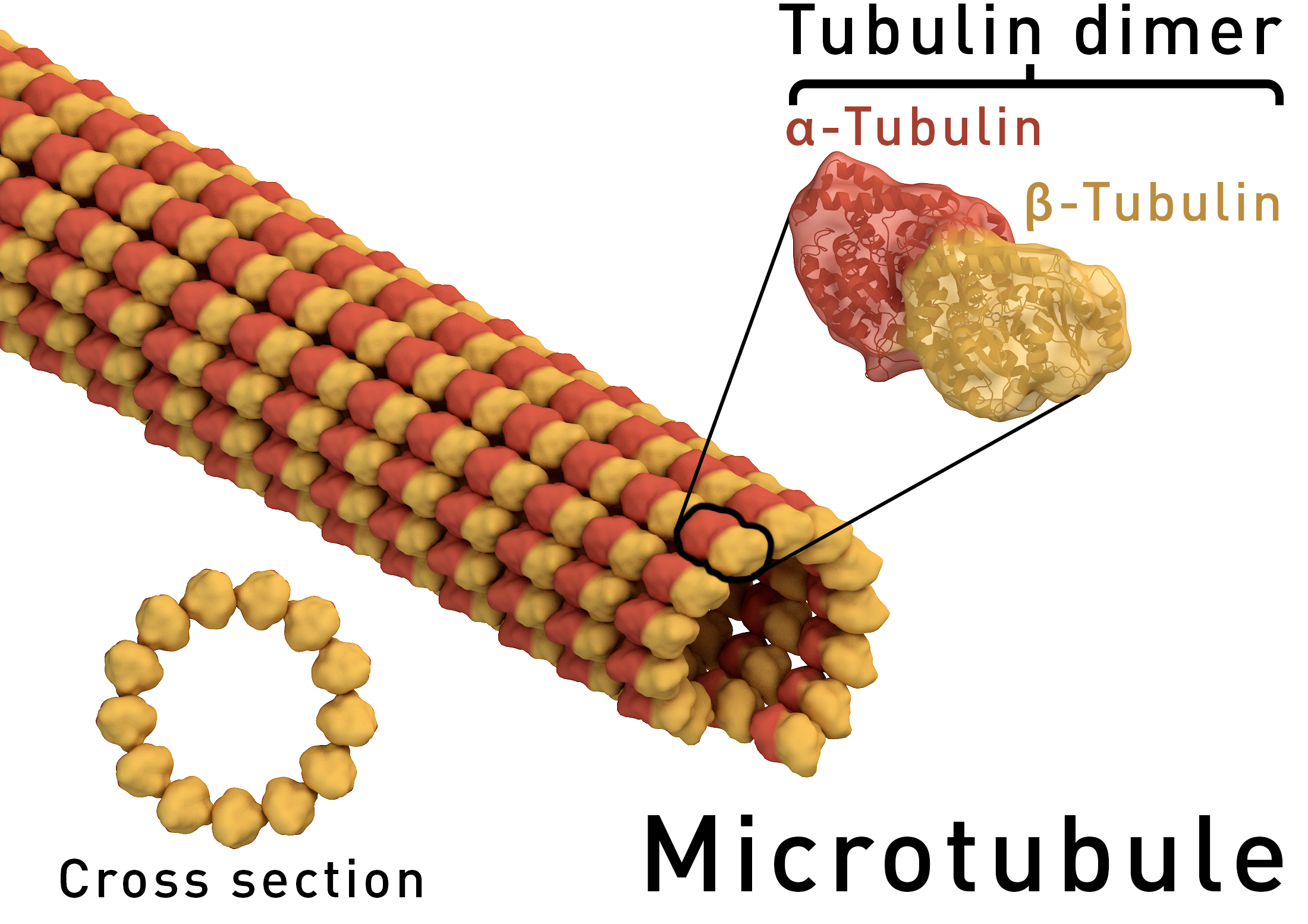
Source: [4]. Licence: CC-BY-SA-4.0.
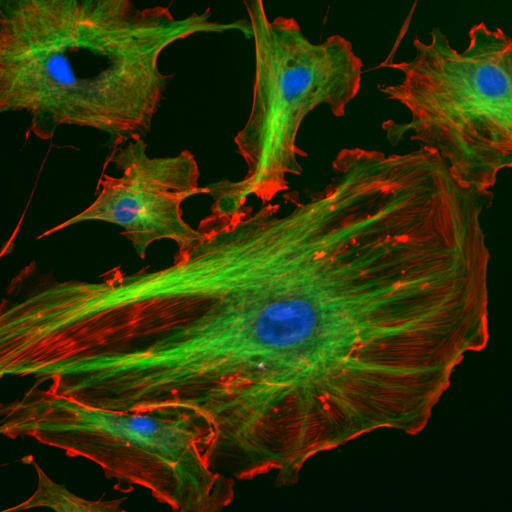
Source: [5]. Licence: CC-BY-SA-4.0.
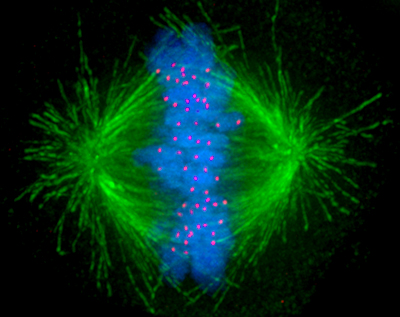
Source: [6]. Licence: none (public domain).
Several families of proteins help build or support microtubules, and these proteins are highly critical for brain development. Loss-of-function mutations in their genes are the cause of many cases of primary microcephaly (microcephaly without any other significant symptoms),[7] and they are implicated in Alzheimer’s disease (e.g., microtubule-associated protein tau), schizophrenia (e.g., disrupted in schizophrenia 1) and autism spectrum disorder.[1][2]
Centrosome
Some microtubule-related proteins achieve their roles via activities at the centrosome, the cellular structure that microtubules are built and anchored from in animals.
Centrosomes contain two centrioles at right-angles to each other, the mother centriole and daughter centriole.[8]
Source: [9]. Licence: CC-BY-3.0.
Cilia
Another role for microtubules is in forming cilia. Cilia are small appendages on cells that come in two forms:[10]
- Motile cilia are found in multiple numbers on a few select groups of cells, such as those in the lungs, Fallopian tubes or ventricles of the brain, and they function by making repetitive beating motions to move fluids or other contents from one location to another.
- Primary cilia are found alone on almost every cell, and they function as small signalling ‘antennae’, containing receptors for proteins or other molecules that guide cell migration, division or differentiation.
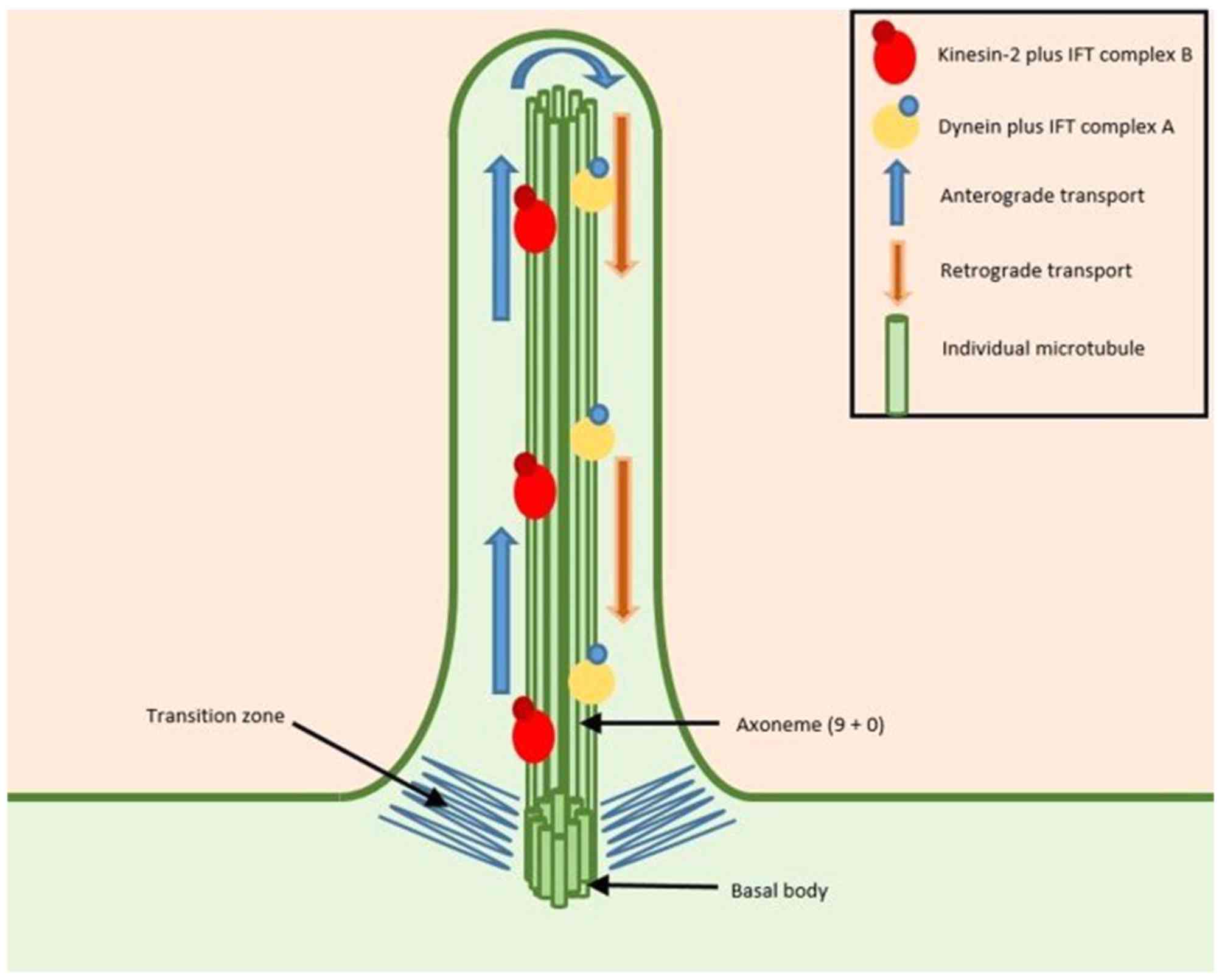
Source: [11]. Licence: CC-BY-NC-ND-4.0.
Cell division and migration can also be impaired via dysfunction in these cilia. Conditions resulting from mutations impairing the development or function of cilia are termed ciliopathies.[12]
NBPF family
The NBPF family is a family of around 23 centrosomal genes in humans that underwent significant expansion in the ancestral human lineage and have been linked to brain size.
In humans, they are found primarily on chromosome 1, in the unstable region 1q21.1–1q21.2, with several copies also found at 1p36, 1p13.3 and 1p12.[13]
Olduvai domain
All of the genes of the NBPF family contain copies of a domain (an independent functional genetic element often found in multiple genes) known as the Olduvai domain (previously known as DUF1220 or the NBPF repeat before 2018[14]), with some containing dozens of repeats of the domain.
The Olduvai domain is only found in the NBPF genes and PDE4DIP (also located at chromosome 1q21.2), from which the NBPF family is determined to have originated by duplication.[13]
PDE4DIP itself was duplicated from CDK5RAP2 (located at chromosome 9q33.2),[15] which has also been linked to brain size (especially in males and especially in the frontal cortex[16]) and causes a form of autosomal recessive primary microcephaly when both copies are mutated.[17]
Subtypes
There are six related versions, or subtypes, of the Olduvai domain.
In humans, the majority of the repeats of the Olduvai domain are a sequence of three related subtypes that originated in Homininae (gorillas, chimpanzees and humans). These subtypes were termed HLS1, HLS2 and HL3 (for human-lineage-specific [increases]) by O’Bleness et al. in 2012.[13]
The HLS triplet contains 6 exons (coding sequences) and 6 introns (non-coding sequences), with the exons comprising 10% of the length of the triplet pattern.[13]
Three other subtypes exist, which were termed CON1, CON2 and CON3 (for conserved).[13]
Despite the multiple genes and domains, in 2006,[18] the genes were only detected to produce copies of a single protein product estimated to be able to contain 3–4 Olduvai domains, which was suggested to represent the final functional form in humans.[19]
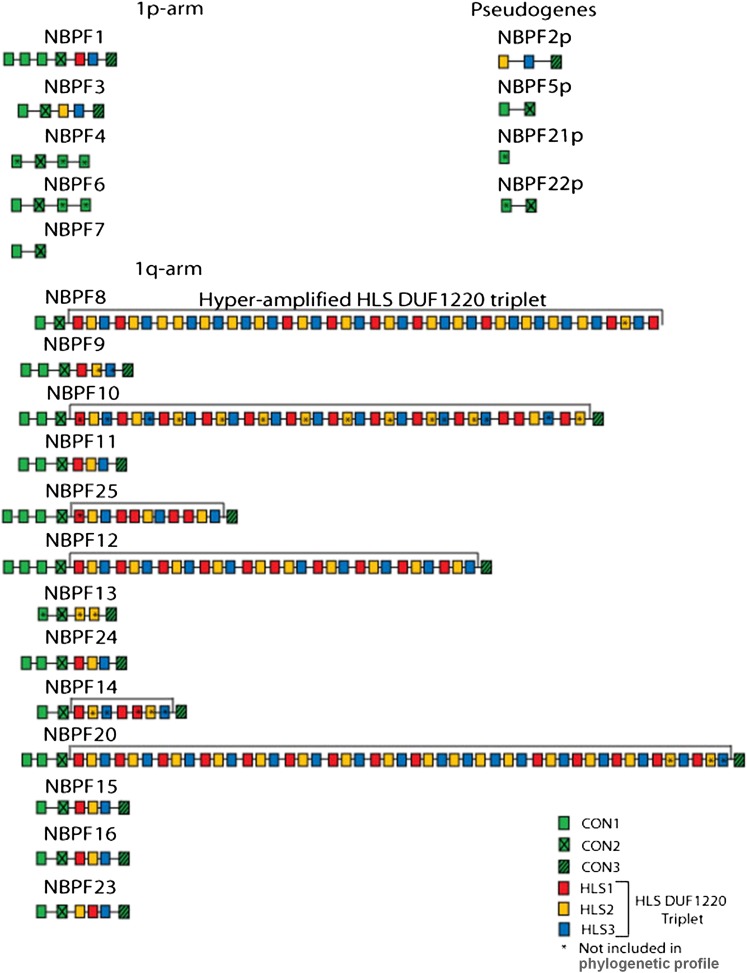
Source: [13]. Licence: CC-BY-3.0.
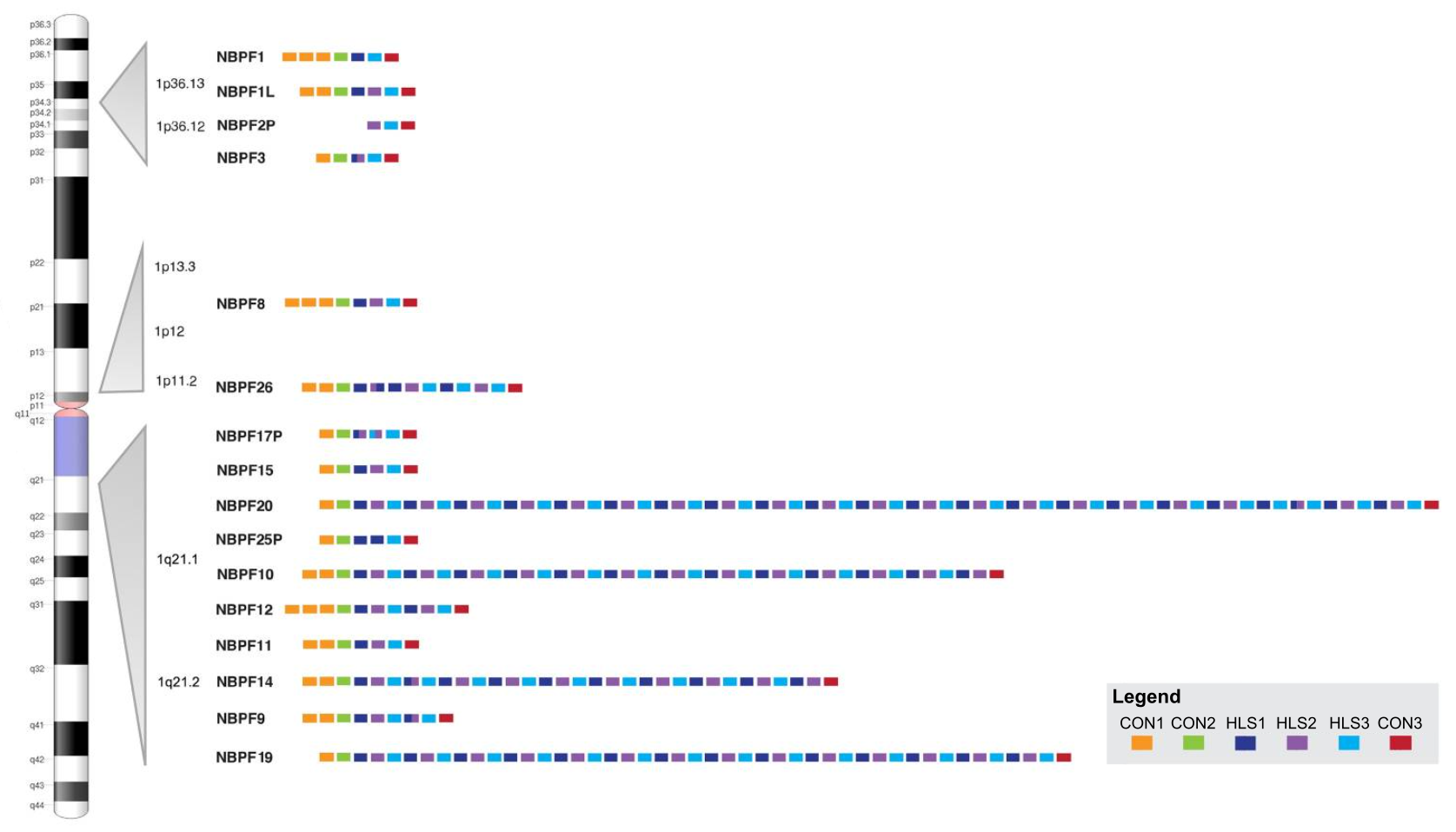
Source: [20]. Licence: CC-BY-4.0.
Roles
NBPF genes code for centrosomal proteins that have roles in brain development and neurogenesis by affecting microtubules.[13]
It has not yet been established in literature how the Olduvai domains contributed specifically to the development of von Economo neurons, however I propose the most likely hypothesis below.
Neurogenesis
The neural tube is the precursor of the brain and spinal cord and forms at week 4 of gestation.[21]
In early development, neurogenesis takes place in the ventricular and subventricular zones (or the germinal matrix), located in the walls of the lateral ventricles. This region is active between weeks 8 and 36 of gestation, peaking at week 25.[22]
Neuroepithelial cells divide symmetrically to increase in number before eventually dividing asymmetrically to mark the beginning of migration and differentiation into neurons and glia.[23]
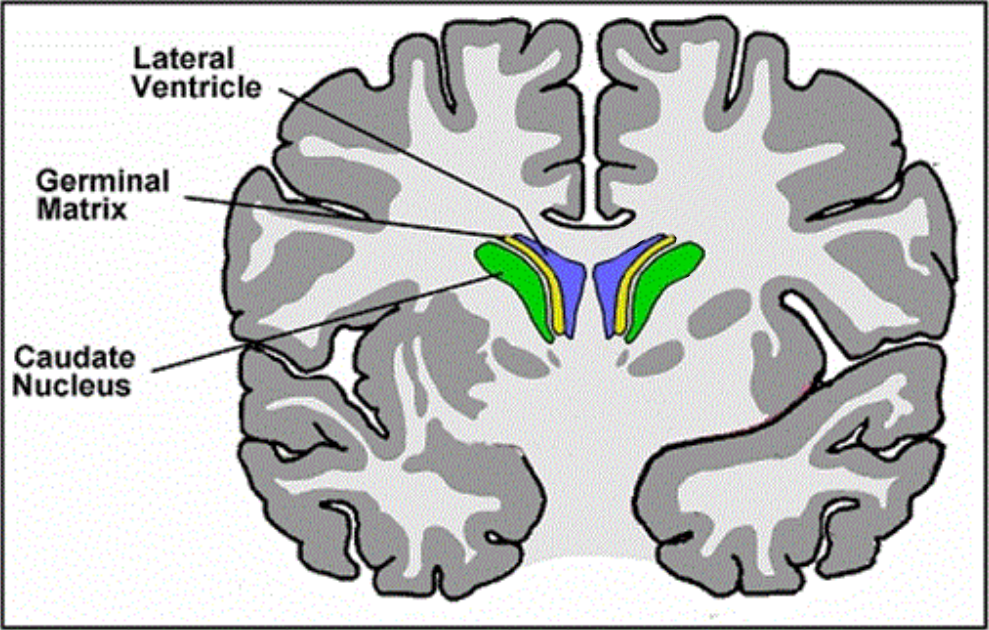
Source: [24]. Licence: CC-BY-4.0.
NBPF genes are strongly expressed in the germinal matrix.[25] NBPF proteins are believed to promote increased symmetric proliferative division of the neuroepithelial cells before their asymmetric cell division and subsequent differentiation and migration.[19]
This has been suggested to involve orientation of the centrosome,[19] similar to other microtubule-related proteins that affect symmetric division through orientation of the microtubular mitotic spindles, such as LIS1, which causes lissencephaly (lack of ridges and grooves in the brain) when both copies of its genes are mutated.[26]
NBPF genes are specifically expressed in neurons rather than glia.[19]
Ciliogenesis
NBPF1 is known to interact with CBY1, a protein that localises at the end of the mother centriole and is essential for primary cilium formation. Its interaction was found to be specifically involved in CBY1’s role as a scaffold for primary cilium formation at the centriole.[19]
NBPF1 was theorised to bind to CBY1 in order to delay primary cilium formation (which would otherwise cause the cell to enter the quiescent (G0), non-dividing state), meaning the cells could divide for longer.[19]
This is an effect similar to that for the other genes duplicated after the evolutionary event discussed below, in which the duplicated genes inhibit and delay the processes that their ancestor genes facilitate (in one of those cases, by its protein binding to the ancestor gene’s protein).
While NBPF1 inhibits cilium formation from the centriole, the gene that the NBPF genes were duplicated from, PDE4DIP, functions to aid in nucleation of microtubules from the centrosome, though PDE4DIP itself can be compensated for by its own paralogue, CDK5RAP2, which is necessary for microtubule nucleation.[27]
Von Economo neurons
Like a few other genes duplicated after the evolutionary event ~3.5 million years ago, the Olduvai domain binds to or otherwise inhibits the function of other proteins (in this case, centrosomal proteins), and this allows the developmental processes that occur during that time to be greatly prolonged and heightened. This may include binding to its similarly-sequenced ancestor, PDE4DIP, but this has not yet been investigated in literature to my knowledge.
How this applies specifically to the brainstem projection neurons of the anterior insula and ventral anterior cingulate cortex has yet to be researched. However, it is likely that, constituting core regions of the cortex close to the brainstem as opposed to external regions, these regions are more strongly affected by the delay in development imposed by the HLS triplets of the Olduvai domain.
The CON copies, which are not in triplet form and have been shown to have a higher contribution per-copy to brain size, likely have a more functional than inhibiting role in comparison to the HLS triplets, similar to the single (or few-copy) Olduvai domain in the PDE4DIP protein.
However, the CON subtypes also correlate with the few other animals with larger brain size than humans and a basal social mindset, and they likely also achieve this by binding and inhibition of other centrosomal proteins, potentially including the similarly-sequenced PDE4DIP.
The transcription factors and other identity markers for the would-be von Economo neurons would have already been in place, and it is likely that the Olduvai domain did not arrive to serve as a marker of these neurons but rather prolonged brain development in a way that especially affected the time that they are formed.
This inhibitory dynamic is likely also responsible for the size and elongated shape of the von Economo neurons; the SRGAP2C gene also created by the evolutionary event, which prolongs the development window of dendritic spines by binding to and inhibiting its ancestor protein SRGAP2, serves to elongate the spines.
In essence, the inhibition by the HLS tripets likely allows for a pooling of more resources into the single neuron, the single dendrite and the single axon at the relative expense of moving on to the formation of other dendrites or pyramidal neurons (or even other cortical layers, as the anterior insula has fewer than the typical six).
Similarly, it likely allows for a pooling of more resources into the earlier-formed, core regions of the cortex closer to the brainstem at the relative (but not absolute) expense of the outer regions of the cortex.
All of this likely resulted in the ability for brainstem signals from the insular cortex to ‘leak’ into the prefrontal cortex via existing but formerly undeveloped routes, diluting it with these continual ‘markers’ that were attached to episodic memories as they formed.
Other existing routes close to the brainstem, such as the ventral anterior cingulate cortex, were also heightened by the HLS-triplet hyperamplification, and since the ‘markers’ in the medial prefrontal cortex were the same signals of the brainstem responses themselves, this would have allowed access of episodic memories to also trigger these brainstem responses via these existing output routes.
Essentially, via its growth and transformations of the neurons in these regions, the Olduvai domains likely formed a clean loop from the brainstem back to the brainstem via the anterior insular cortex, the medial prefrontal cortex and the anterior cingulate cortex, completing a new functional circuit in the brain.
Evolution
The first Olduvai domain appeared in PDE4DIP in mammals at least 200 million years ago, while PDE4DIP itself has existed as far back as bony fish, 450 million years ago.[13]
The NBPF family resulted from a series of duplication events, beginning with the duplication of PDE4DIP into the first NBPF gene in Eutheria, the stem group of placental mammals, roughly 100–150 million years ago.[13]
Several lineages, such as most of Afrotheria (except elephants), Xenarthra (sloths, armadillos, etc.), Chiroptera (bats), Eulipotyphla (hedgehogs, shrews, etc.) and Rodentia (rodents), later lost this gene.[13]
In some Boreoeutherians (such as cat, horse, cow), the first NBPF gene underwent minor duplication into 2 or 3 copies. In some (such as dog, horse, dolphin), the Olduvai domain within the NBPF gene(s) underwent duplication into a few copies.[13]
Beginning in the simian infraorder (Simiiformes) of primates, which includes all monkeys, apes and humans, the NBPF family and its Olduvai domains both underwent progressive duplication until reaching ~23 NBPF genes and suddenly reaching ~270–300 Olduvai domains in humans after the split from chimpanzees.[13][28]
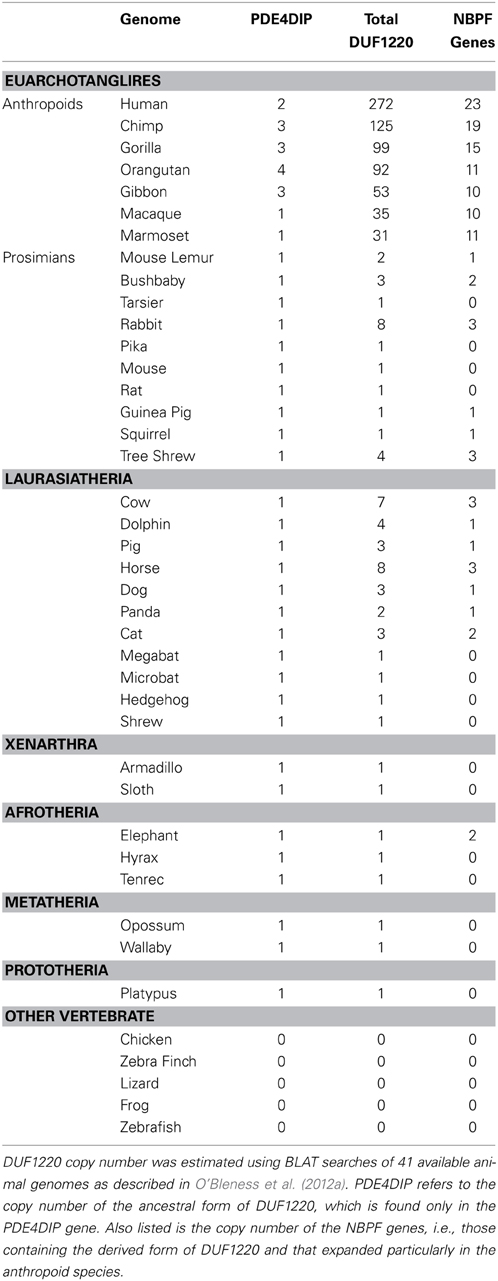
Source: [19]. Licence: CC-BY-3.0.
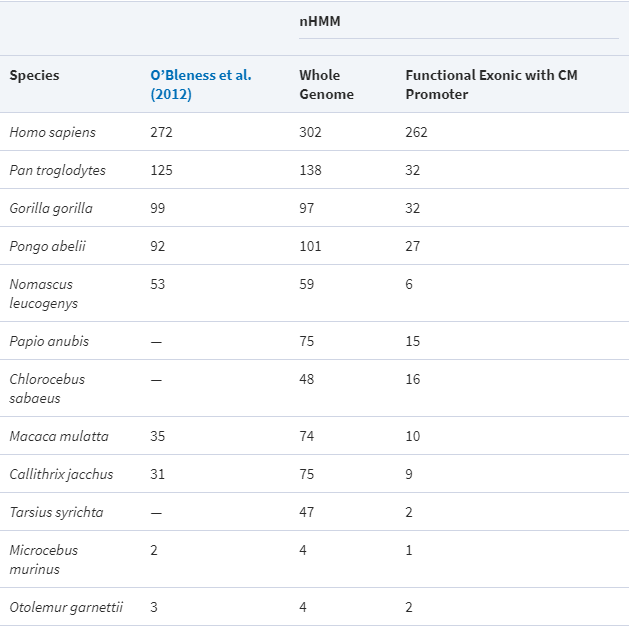
Source: [28]. Licence: CC-BY-NC-4.0.
Neanderthals, which diverged from ancestors of early humans around 600,000 years ago,[29] had a larger brain volume than modern humans.[30] In one study, they were reported to have had ~350 Olduvai copies,[19] however in another study, they were reported to have had ~272 (the same number of domains and genes as in a human genome).[31]
Pericentric inversion
The hyperamplification of the HLS Olduvai domains in humans was the result of a pericentric inversion (in which the region around a chromosome’s centromere inverts) that occurred between 1p11.2 and 1q21.2 in the human lineage around 3.5 million years ago, after the separation from chimpanzees around 7 million years ago, which also affected many other genes and led to this region being unstable to this day.[13]
Pairs of chromosomes in which one contains a pericentric inversion and the other does not (a form of heterozygosity) have difficulties in recombination during meiosis, which can lead to non-allelic homologous recombination, in which deletions and duplications are much more propense to occur. This, combined with the fact that higher copies of Olduvai domains would have had an evolutionary advantage, was theorised to have resulted in the rapid duplication and persistence of Olduvai domains in humans.[13]
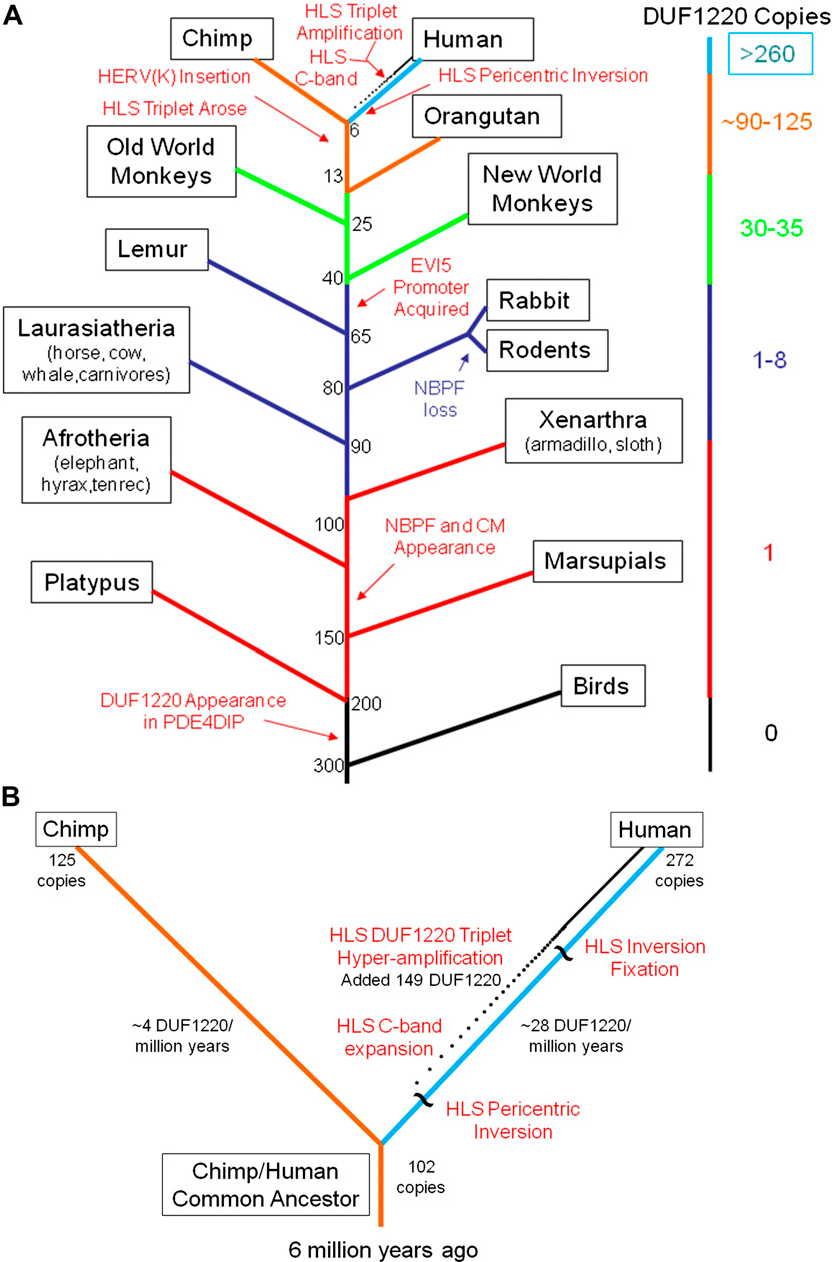
B: Zoomed-in view of the human and chimpanzee regions of the tree, showing the increasing accumulation (dotted line) of around 28 Olduvai copies every million years in the human lineage following the pericentric inversion.
Source: [13]. Licence: CC-BY-3.0.
Evolution of social-mindset features
The genus of Homo originated from a lineage of Australopithecus around 3 million years ago and was traditionally conceived to mark the beginning of tool cultures (the Oldowan culture of Olduvai Gorge, from which the Olduvai domain now gets its name[14]), although there is now evidence of tool use in Australopithecus.[32]
There was some 43% of an increase in intracranial volume between late Australopithecus (as a reference, Australopithecus garhi of 2.5 million years ago: 460 cm³) and early Homo (Homo habilis of 2–3 million years ago: 660 cm³).[33]
Homo erectus, which split from Homo habilis around 2 million years ago and lasted until 100,000 years ago in Asia, is the first archaic human species known to manipulate fire[34] and possibly engage in art.[35][36] Its cranial capacity ranged from around 813–1251 cm³ (an increase of around 23–89% from Homo habilis), though early Georgian specimens of Homo georgicus/Homo erectus georgicus, which is sometimes not regarded as Homo erectus,[37] had a cranial capacity as low as 543 cm³.[38]
In the other main lineage (in Africa) after Homo habilis, intracranial volume also increased by around 89% to around 1,250 cm³ in Homo heidelbergensis of around 700,000 years ago, often considered to be the direct ancestor of modern Homo sapiens. Homo heidelbergensis also shares a common ancestor with (or is the ancestor of) Neanderthals and Denisovans, which split at around the same time and migrated to Eurasia[39] while Homo sapiens remained in Africa.
Modern human intracranial volume is around 1,270 cm³ for males and 1,130 cm³ for females.[40]
It is well established that Neanderthals had advanced communication and cultural features similar to modern humans, such as potentially articulate directed language,[41] regional cultures,[42] clothing,[43] art and personal adornment[44] and collection of unusual crystals or fossils.[45] Claims of religious beliefs have been made, most directly evidenced by burial rituals.[46] Both Neanderthals and Denisovans extensively interbred with Homo sapiens, which would likely not have been possible without these similarities.
For reference, the oldest known still-existing direct paternal or maternal lineage among current humans (as of September 2020) is Y haplogroup A00 (around 250,000 years old), found in a portion of the populations of ethnic groups of western Cameroon,[47] and the current populations of humans outside Africa, as well as most of the current populations of humans of Africa, originated from migrations that began around 70,000 years ago (with Y haplogroups DE and CF and mtDNA haplogroup L3).
It is highly likely that the hyperamplification of the HLS Olduvai domains and the resulting human social mindset evolutionarily pressured the loss of the oestrous cycle, the visible and aural exaggeration of existing brainstem-response mechanisms for laughing/crying (to include tears, for example[48]), the loss of human body hair (due to greater flea transmission[49][50] or use of fire[51]) and several other characteristically human physiological features.
Clinical significance in high-functioning autism
A 2015 study examined repeat numbers of the HLS1 Olduvai subtype in a sample of those with autism spectrum disorder (mostly high-functioning with average intelligence), with HLS1 being used as a proxy for the HLS1–3 triplet.[52]
All in the sample had the repeats in the normal range (HLS1: 125–257, mean 198), however HLS1 repeat number was found to be associated with reduced severity of social deficits. The sample almost entirely contained cases of autism without intellectual disability, as opposed to a previous sample in which this relationship was not found.[52]
A 2019 study assessed repeat numbers of the HLS1 Olduvai subtype in a sample of those with autism spectrum disorder, again using HLS1 as a proxy for the HLS1–3 triplet. The mean HLS1 copy number of the sample was 200.[53]
HLS1 copy number was found to be correlated with lower communication-impairment score. The associations were only found in inherited/familial ASD and not de novo ASD (with likely intellectual disability).[53]
Other genes created by the pericentric inversion
The NBPF family existed before the human pericentric inversion between chromosome 1p11.2 and 1q21.1 and was merely hyperamplified by it. When adjusting for brain size (due to other genetic contributors), they correlate with the few other animals with a basal social mindset or von Economo neurons, such as bonobos, dolphins or elephants.
Below are two groups of genes that were created only in the human lineage by the pericentric inversion between chromosome 1p11.2 and 1q21.1. Neither are functionally present in the few other animals with a basal social mindset or von Economo neurons, but they are mentioned here for completeness.
NOTCH2NLA, NOTCH2NLB and NOTCH2NLC
Notch is a well studied, highly conserved juxtacrine signalling pathway through which a variety of developmental processes occur, including brain development. The four Notch receptors are NOTCH1, NOTCH2, NOTCH3 and NOTCH4.[54]
Three NBPF genes that contain the hyperamplified HLS Olduvai copies, NBPF10, NBPF14 and NBPF19, were duplicated in combination with new NOTCH genes adjacent to each gene.
Other Homininae (gorillas and chimpanzees) have the non-functional, truncated pseudogene NOTCH2NLR (‘R’ for ‘related’) adjacent to PDE4DIP at 1q21.1.[55]
In the human lineage, some time before the pericentric inversion of chromosome 1 around 3.5 million years ago, the promoter (the sequence that initiates transcription) and first exon of NOTCH2, located at chromosome 1p12, was pasted onto NOTCH2NLR in a gene conversion event, rendering NOTCH2NLR temporarily functional.[55]
Following the pericentric inversion, NOTCH2NLR and NBPF26 duplicated into NOTCH2NLB and NBPF14, which moved to locus 1q21.1.[20] Later, NOTCH2NLB and NBPF14 duplicated into NOTCH2NLA and NBPF10 as well as NOTCH2NLC and NBPF19, also at 1q21.1.[20]
NOTCH2NLR appears to be non-functional once again in humans and is not found in 14% of people.[55]
In conjunction with the roles of the NBPF genes, the NOTCH2NL genes delay neuronal differentiation.[55] NOTCH2NLB is the most expressed NOTCH2NL gene, followed closely by NOTCH2NLA, with NOTCH2NLC being less expressed.[56]
SRGAP2C
The pericentric inversion at 1p11.2 and 1q21.1 also led to the duplication of another gene, SRGAP2 (SLIT-ROBO Rho GTPase activating protein). Its promoter and first nine exons were duplicated into SRGAP2B at 1q21.1 roughly 3.4 million years ago, which was then duplicated into SRGAP2C at 1p12 roughly 2.4 million years ago and SRGAP2D at 1q21.1 roughly 1 million years ago.[57]
SRGAP2C is considered to be the only active new gene. The other two new genes are highly variable in their number of copies among humans, including not being present at all.[58]
SRGAP2 expression is increased at the end of cortical neuron migration and is involved in terminating the period of radial migration of neurons and moving through the dendritic-spine development phase. SRGAP2C binds to and inhibits the function of SRGAP2, extending the distance by which neurons radially migrate and prolonging the dendritic-spine development period, leading to increased density of longer dendritic spines.[59]
Loss-of-function mutations in the ancestral SRGAP2 gene lead to an infantile epileptic encephalopathy.[60]
References
- ^ a b Lasser, Micaela; Tiber, Jessica; Lowery, Laura Anne (2018). "The Role of the Microtubule Cytoskeleton in Neurodevelopmental Disorders". Frontiers in Cellular Neuroscience. 12. doi:10.3389/fncel.2018.00165. ISSN 1662-5102.
- ^ a b Chang, Qiaoqiao; Yang, Hua; Wang, Min; Wei, Hongen; Hu, Fengyun (2018-06-23). "Role of Microtubule-Associated Protein in Autism Spectrum Disorder". Neuroscience Bulletin. 34 (6): 1119–1126. doi:10.1007/s12264-018-0246-2. ISSN 1673-7067. PMC 6246838. PMID 29936584.
- ^ Etienne‐Manneville, Sandrine (2004). "Actin and Microtubules in Cell Motility: Which One is in Control?" Traffic. 5 (7): 470–477. doi:10.1111/j.1600-0854.2004.00196.x. ISSN 1600-0854.
- ^ Splettstoesser, Thomas (2015-06-16). "English: Diagram showing a microtubule and the alpha/beta-tubulin heterodimer it's being constructed from." Wikimedia Commons.
- ^ Splette (2006-03-24). "Endothelial cells seen under a microscope. In blue, nuclei marked with DAPI. In green, microtubules marked with an antibody. In red, actin labeled with phalloidin." Wikimedia Commons.
- ^ Afunguy (2006-10-13). "English: Image of the mitotic spindle in a human cell showing microtubules in green, chromosomes (DNA) in blue, and kinetochores in red." Wikimedia Commons.
- ^ Thornton, Gemma K.; Woods, Geoffrey (2009-11-01). "Primary microcephaly: do all roads lead to Rome?" Trends in Genetics. 25 (11): 501–510. doi:10.1016/j.tig.2009.09.011. ISSN 0168-9525.
- ^ Schatten, Heide (2008-6). "The mammalian centrosome and its functional significance". Histochemistry and Cell Biology. 129 (6): 667–686. doi:10.1007/s00418-008-0427-6. ISSN 0948-6143. PMC 2386528. PMID 18437411.
- ^ Kelvinsong (2012-12-09). "English: Diagram of a centrosome, without the yellow frame." Wikimedia Commons.
- ^ Jain, Raksha; Javidan-Nejad, Cylen; Alexander-Brett, Jennifer; Horani, Amjad; Cabellon, Michelle C.; Walter, Michael J.; Brody, Steven L (2012-01-01). "Sensory functions of motile cilia and implication for bronchiectasis". Frontiers in bioscience (Scholar edition). 4: 1088–1098. ISSN 1945-0516. PMC 3841983. PMID 22202111.
- ^ Higgins, Michael; Obaidi, Ismael; McMorrow, Tara (2019-03-01). "Primary cilia and their role in cancer (Review)". Oncology Letters. 17 (3): 3041–3047. doi:10.3892/ol.2019.9942. ISSN 1792-1074.
- ^ Badano, Jose L.; Mitsuma, Norimasa; Beales, Phil L.; Katsanis, Nicholas (2006-09-01). "The Ciliopathies: An Emerging Class of Human Genetic Disorders". Annual Review of Genomics and Human Genetics. 7 (1): 125–148. doi:10.1146/annurev.genom.7.080505.115610. ISSN 1527-8204.
- ^ a b c d e f g h i j k l m n o O’Bleness, Majesta S.; Dickens, C. Michael; Dumas, Laura J.; Kehrer-Sawatzki, Hildegard; Wyckoff, Gerald J.; Sikela, James M (2012-09-01). "Evolutionary History and Genome Organization of DUF1220 Protein Domains". G3: Genes|Genomes|Genetics. 2 (9): 977–986. doi:10.1534/g3.112.003061. ISSN 2160-1836. PMC 3429928. PMID 22973535.
- ^ a b Sikela, James M.; van Roy, Frans (2018-07-17). "Changing the name of the NBPF/DUF1220 domain to the Olduvai domain". F1000Research. 6. doi:10.12688/f1000research.13586.2. ISSN 2046-1402. PMC 5773923. PMID 29399325.
- ^ Dumas, Laura; Kim, Young H.; Karimpour-Fard, Anis; Cox, Michael; Hopkins, Janet; Pollack, Jonathan R.; Sikela, James M (2007-9). "Gene copy number variation spanning 60 million years of human and primate evolution". Genome Research. PubMed Central. 17 (9): 1266–1277. doi:10.1101/gr.6557307. ISSN 1088-9051. PMC 1950895. PMID 17666543.
- ^ Rimol, Lars M.; Agartz, Ingrid; Djurovic, Srdjan; Brown, Andrew A.; Roddey, J. Cooper; Kähler, Anna K.; Mattingsdal, Morten; Athanasiu, Lavinia; Joyner, Alexander H.; Schork, Nicholas J.; Halgren, Eric; Sundet, Kjetil; Melle, Ingrid; Dale, Anders M.; Andreassen, Ole A (2010-01-05). "Sex-dependent association of common variants of microcephaly genes with brain structure". Proceedings of the National Academy of Sciences of the United States of America. 107 (1): 384–388. doi:10.1073/pnas.0908454107. ISSN 0027-8424. PMC 2806758. PMID 20080800.
- ^ "OMIM Entry - # 604804 - MICROCEPHALY 3, PRIMARY, AUTOSOMAL RECESSIVE; MCPH3". Online Mendelian Inheritance in Man. (Archive version from 7 October 2020.)
- ^ Popesco, Magdalena C.; MacLaren, Erik J.; Hopkins, Janet; Dumas, Laura; Cox, Michael; Meltesen, Lynne; McGavran, Loris; Wyckoff, Gerald J.; Sikela, James M (2006-09-01). "Human Lineage–Specific Amplification, Selection, and Neuronal Expression of DUF1220 Domains". Science. science.sciencemag.org. 313 (5791): 1304–1307. doi:10.1126/science.1127980. ISSN 0036-8075, 1095-9203. PMID 16946073.
- ^ a b c d e f g h Keeney, Jonathon G.; Dumas, Laura; Sikela, James M (2014). "The case for DUF1220 domain dosage as a primary contributor to anthropoid brain expansion". Frontiers in Human Neuroscience. 8. doi:10.3389/fnhum.2014.00427. ISSN 1662-5161.
- ^ a b c Fiddes, Ian T.; Pollen, Alex A.; Davis, Jonathan M.; Sikela, James M (2019). "Paired involvement of human-specific Olduvai domains and NOTCH2NL genes in human brain evolution". Human Genetics. 138 (7): 715–721. doi:10.1007/s00439-019-02018-4. ISSN 0340-6717. PMC 6611739. PMID 31087184.
- ^ Singh, Ranbir; Munakomi, Sunil (2020). "Embryology, Neural Tube". PMID 31194425.
- ^ Raets, M. M. A.; Dudink, J.; Govaert, P (2015-11-20). "Neonatal disorders of germinal matrix". The Journal of Maternal-Fetal & Neonatal Medicine. 28 (sup1): 2286–2290. doi:10.3109/14767058.2013.796169. ISSN 1476-7058. PMID 23968365.
- ^ Götz, Magdalena; Huttner, Wieland B (2005-10). "The cell biology of neurogenesis". Nature Reviews Molecular Cell Biology. 6 (10): 777–788. doi:10.1038/nrm1739. ISSN 1471-0080.
- ^ Talbert, David (2016). "Cerebral Venous Malformation as a Cause of Neonatal Intra-Ventricular Haemorrhage and Unexplained Infant Subdural Haemorrhage". Anatomy & Physiology: Current Research. Longdom Publishing SL. 6 (2). doi:10.4172/2161-0940.1000202. ISSN 2161-0940. (Image edited by myself.)
- ^ Snuderl, Matija; Kannan, Kasthuri; Pfaff, Elke; Wang, Shiyang; Stafford, James M.; Serrano, Jonathan; Heguy, Adriana; Ray, Karina; Faustin, Arline; Aminova, Olga; Dolgalev, Igor; Stapleton, Stacie L.; Zagzag, David; Chiriboga, Luis; Gardner, Sharon L.; Wisoff, Jeffrey H.; Golfinos, John G.; Capper, David; Hovestadt, Volker; Rosenblum, Marc K.; Placantonakis, Dimitris G.; LeBoeuf, Sarah E.; Papagiannakopoulos, Thales Y.; Chavez, Lukas; Ahsan, Sama; Eberhart, Charles G.; Pfister, Stefan M.; Jones, David T. W.; Karajannis, Matthias A (2018-07-20). "Recurrent homozygous deletion of DROSHA and microduplication of PDE4DIP in pineoblastoma". Nature Communications. 9 (1): 1–10. doi:10.1038/s41467-018-05029-3. ISSN 2041-1723.
- ^ Yingling, Jessica; Youn, Yong Ha; Darling, Dawn; Toyo-oka, Kazuhito; Pramparo, Tiziano; Hirotsune, Shinji; Wynshaw-Boris, Anthony (2008-02-08). "Neuroepithelial Stem Cell Proliferation Requires LIS1 for Precise Spindle Orientation and Symmetric Division". Cell. 132 (3): 474–486. doi:10.1016/j.cell.2008.01.026. ISSN 0092-8674, 1097-4172. PMID 18267077.
- ^ Roubin, Régine; Acquaviva, Claire; Chevrier, Véronique; Sedjaï, Fatima; Zyss, Déborah; Birnbaum, Daniel; Rosnet, Olivier (2012-12-18). "Myomegalin is necessary for the formation of centrosomal and Golgi-derived microtubules". Biology Open. PubMed Central. 2 (2): 238–250. doi:10.1242/bio.20123392. ISSN 2046-6390. PMC 3575658. PMID 23430395.
- ^ a b Zimmer, Fabian; Montgomery, Stephen H (2015-08-01). "Phylogenetic Analysis Supports a Link between DUF1220 Domain Number and Primate Brain Expansion". Genome Biology and Evolution. 7 (8): 2083–2088. doi:10.1093/gbe/evv122.
- ^ Green, Richard E.; Malaspinas, Anna-Sapfo; Krause, Johannes; Briggs, Adrian W.; Johnson, Philip L. F.; Uhler, Caroline; Meyer, Matthias; Good, Jeffrey M.; Maricic, Tomislav; Stenzel, Udo; Prüfer, Kay; Siebauer, Michael; Burbano, Hernán A.; Ronan, Michael; Rothberg, Jonathan M.; Egholm, Michael; Rudan, Pavao; Brajković, Dejana; Kućan, Željko; Gušić, Ivan; Wikström, Mårten; Laakkonen, Liisa; Kelso, Janet; Slatkin, Montgomery; Pääbo, Svante (2008-08-08). "A complete Neandertal mitochondrial genome sequence determined by high-throughput sequencing". Cell. 134 (3): 416–426. doi:10.1016/j.cell.2008.06.021. ISSN 0092-8674. PMC 2602844. PMID 18692465.
- ^ León, Marcia S. Ponce de; Golovanova, Lubov; Doronichev, Vladimir; Romanova, Galina; Akazawa, Takeru; Kondo, Osamu; Ishida, Hajime; Zollikofer, Christoph P. E (2008-09-16). "Neanderthal brain size at birth provides insights into the evolution of human life history". Proceedings of the National Academy of Sciences. www.pnas.org. 105 (37): 13764–13768. doi:10.1073/pnas.0803917105. ISSN 0027-8424, 1091-6490. PMID 18779579.
- ^ Perez, Jean-Claude (2017-08-07). "DUF1220 Homo Sapiens and Neanderthal fractal periods architectures breakthrough". SDRP Journal of Cellular and Molecular Physiology. 1 (1): 25–49. doi:http://dx.doi.org/10.25177/JCMP.1.1.4. ISSN 2574-4046.
- ^ McPherron, Shannon P.; Alemseged, Zeresenay; Marean, Curtis W.; Wynn, Jonathan G.; Reed, Denné; Geraads, Denis; Bobe, René; Béarat, Hamdallah A (2010-08-12). "Evidence for stone-tool-assisted consumption of animal tissues before 3.39 million years ago at Dikika, Ethiopia". Nature. 466 (7308): 857–860. doi:10.1038/nature09248. ISSN 1476-4687. PMID 20703305.
- ^ Kimbel, William H.; Villmoare, Brian (2016-07-05). "From Australopithecus to Homo: the transition that wasn't†". Philosophical Transactions of the Royal Society B: Biological Sciences. 371 (1698). doi:10.1098/rstb.2015.0248. ISSN 0962-8436. PMC 4920303. PMID 27298460.
- ^ Gowlett, J. A. J (2016-06-05). "The discovery of fire by humans: a long and convoluted process". Philosophical Transactions of the Royal Society B: Biological Sciences. 371 (1696). doi:10.1098/rstb.2015.0164. ISSN 0962-8436. PMC 4874402. PMID 27216521.
- ^ Dickson, D. Bruce (1992). "The Dawn of Belief: Religion in the Upper Paleolithic of Southwestern Europe".
- ^ Joordens, Josephine C. A.; d’Errico, Francesco; Wesselingh, Frank P.; Munro, Stephen; de Vos, John; Wallinga, Jakob; Ankjærgaard, Christina; Reimann, Tony; Wijbrans, Jan R.; Kuiper, Klaudia F.; Mücher, Herman J.; Coqueugniot, Hélène; Prié, Vincent; Joosten, Ineke; van Os, Bertil; Schulp, Anne S.; Panuel, Michel; van der Haas, Victoria; Lustenhouwer, Wim; Reijmer, John J. G.; Roebroeks, Wil (2015-02). "Homo erectus at Trinil on Java used shells for tool production and engraving". Nature. 518 (7538): 228–231. doi:10.1038/nature13962. ISSN 1476-4687.
- ^ Guimarães, Santiago Wolnei Ferreira; Merino, Carlos Lorenzo (2015). "Dmanisi hominin fossils and the problem of the multiple species in the early Homo genus". The Canadian Student Journal of Anthropology. 23 (2).
- ^ Antón, Susan C.; Taboada, Hannah G.; Middleton, Emily R.; Rainwater, Christopher W.; Taylor, Andrea B.; Turner, Trudy R.; Turnquist, Jean E.; Weinstein, Karen J.; Williams, Scott A (2016-07-05). "Morphological variation in Homo erectus and the origins of developmental plasticity". Philosophical Transactions of the Royal Society B: Biological Sciences. 371 (1698). doi:10.1098/rstb.2015.0236. ISSN 0962-8436. PMC 4920293. PMID 27298467.
- ^ Buck, Laura T.; Stringer, Chris B (2014-03-17). "Homo heidelbergensis". Current Biology. 24 (6): R214–R215. doi:10.1016/j.cub.2013.12.048. ISSN 0960-9822. PMID 24650901.
- ^ Allen, John S.; Damasio, Hanna; Grabowski, Thomas J (2002). "Normal neuroanatomical variation in the human brain: An MRI-volumetric study". American Journal of Physical Anthropology. 118 (4): 341–358. doi:10.1002/ajpa.10092. ISSN 1096-8644.
- ^ Dediu, Dan; Levinson, Stephen C (2018-06-01). "Neanderthal language revisited: not only us". Current Opinion in Behavioral Sciences. 21: 49–55. doi:10.1016/j.cobeha.2018.01.001. ISSN 2352-1546.
- ^ Ruebens, Karen (2013-10-01). "Regional behaviour among late Neanderthal groups in Western Europe: A comparative assessment of late Middle Palaeolithic bifacial tool variability". Journal of Human Evolution. 65 (4): 341–362. doi:10.1016/j.jhevol.2013.06.009. ISSN 0047-2484.
- ^ Collard, Mark; Tarle, Lia; Sandgathe, Dennis; Allan, Alexander (2016-12-01). "Faunal evidence for a difference in clothing use between Neanderthals and early modern humans in Europe". Journal of Anthropological Archaeology. 44: 235–246. doi:10.1016/j.jaa.2016.07.010. ISSN 0278-4165.
- ^ Hoffmann, Dirk L.; Angelucci, Diego E.; Villaverde, Valentín; Zapata, Josefina; Zilhão, João (2018-02-22). "Symbolic use of marine shells and mineral pigments by Iberian Neandertals 115,000 years ago". Science Advances. 4 (2). doi:10.1126/sciadv.aar5255. ISSN 2375-2548. PMC 5833998. PMID 29507889.
- ^ Moncel, M. -H.; Chiotti, L.; Gaillard, C.; Onoratini, G.; Pleurdeau, D (2012-03-01). "Non-utilitarian lithic objects from the European Paleolithic". Archaeology, Ethnology and Anthropology of Eurasia. 40 (1): 24–40. doi:10.1016/j.aeae.2012.05.004. ISSN 1563-0110.
- ^ "Neanderthal Burials Confirmed as Ancient Ritual". National Geographic News. 2013-12-16. (Archive version from 8 July 2020.)
- ^ Mendez, Fernando L.; Krahn, Thomas; Schrack, Bonnie; Krahn, Astrid-Maria; Veeramah, Krishna R.; Woerner, August E.; Fomine, Forka Leypey Mathew; Bradman, Neil; Thomas, Mark G.; Karafet, Tatiana M.; Hammer, Michael F (2013-03-07). "An African American Paternal Lineage Adds an Extremely Ancient Root to the Human Y Chromosome Phylogenetic Tree". The American Journal of Human Genetics. 92 (3): 454–459. doi:10.1016/j.ajhg.2013.02.002. ISSN 0002-9297, 1537-6605. PMID 23453668.
- ^ Bylsma, Lauren M.; Gračanin, Asmir; Vingerhoets, Ad J. J. M (2019-02-01). "The neurobiology of human crying". Clinical Autonomic Research. 29 (1): 63–73. doi:10.1007/s10286-018-0526-y. ISSN 1619-1560.
- ^ Pagel, Mark; Bodmer, Walter (2003-08-07). "A naked ape would have fewer parasites." Proceedings of the Royal Society B: Biological Sciences. 270 (Suppl 1): S117–S119. doi:10.1098/rsbl.2003.0041. ISSN 0962-8452. PMC 1698033. PMID 12952654.
- ^ Rantala, M. J (1999-12). "Human nakedness: adaptation against ectoparasites?" International Journal for Parasitology. 29 (12): 1987–1989. doi:10.1016/s0020-7519(99)00133-2. ISSN 0020-7519. PMID 10961855.
- ^ Couch, Alan J (2016-02-03). "Fur or Fire: Was the use of fire the initial selection pressure for fur loss in ancestral hominins?" PeerJ PrePrints. doi:https://doi.org/10.7287/peerj.preprints.1702v1.
- ^ a b Davis, JM; Quick, VB Searles; Sikela, JM (2015-6). "Replicated linear association between DUF1220 copy number and severity of social impairment in autism". Human genetics. 134 (6): 569–575. doi:10.1007/s00439-015-1537-6. ISSN 0340-6717. PMC 5886748. PMID 25758905.
- ^ a b Davis, Jonathan M.; Heft, Ilea; Scherer, Stephen W.; Sikela, James M (2019-02-15). "A Third Linear Association Between Olduvai (DUF1220) Copy Number and Severity of the Classic Symptoms of Inherited Autism". American Journal of Psychiatry. 176 (8): 643–650. doi:10.1176/appi.ajp.2018.18080993. ISSN 0002-953X.
- ^ Andersson, Emma R.; Sandberg, Rickard; Lendahl, Urban (2011-09-01). "Notch signaling: simplicity in design, versatility in function". Development. 138 (17): 3593–3612. doi:10.1242/dev.063610. ISSN 0950-1991, 1477-9129. PMID 21828089.
- ^ a b c d Fiddes, Ian T.; Lodewijk, Gerrald A.; Mooring, Meghan; Bosworth, Colleen M.; Ewing, Adam D.; Mantalas, Gary L.; Novak, Adam M.; Bout, Anouk van den; Bishara, Alex; Rosenkrantz, Jimi L.; Lorig-Roach, Ryan; Field, Andrew R.; Haeussler, Maximilian; Russo, Lotte; Bhaduri, Aparna; Nowakowski, Tomasz J.; Pollen, Alex A.; Dougherty, Max L.; Nuttle, Xander; Addor, Marie-Claude; Zwolinski, Simon; Katzman, Sol; Kriegstein, Arnold; Eichler, Evan E.; Salama, Sofie R.; Jacobs, Frank M. J.; Haussler, David (2018-05-31). "Human-Specific NOTCH2NL Genes Affect Notch Signaling and Cortical Neurogenesis". Cell. 173 (6): 1356–1369.e22. doi:10.1016/j.cell.2018.03.051. ISSN 0092-8674, 1097-4172. PMID 29856954.
- ^ Suzuki, Ikuo K.; Gacquer, David; Heurck, Roxane Van; Kumar, Devesh; Wojno, Marta; Bilheu, Angéline; Herpoel, Adèle; Lambert, Nelle; Cheron, Julian; Polleux, Franck; Detours, Vincent; Vanderhaeghen, Pierre (2018-05-31). "Human-Specific NOTCH2NL Genes Expand Cortical Neurogenesis through Delta/Notch Regulation". Cell. 173 (6): 1370–1384.e16. doi:10.1016/j.cell.2018.03.067. ISSN 0092-8674, 1097-4172. PMID 29856955.
- ^ Sporny, Michael; Guez-Haddad, Julia; Kreusch, Annett; Shakartzi, Sivan; Neznansky, Avi; Cross, Alice; Isupov, Michail N.; Qualmann, Britta; Kessels, Michael M.; Opatowsky, Yarden (2017-6). "Structural History of Human SRGAP2 Proteins". Molecular Biology and Evolution. 34 (6): 1463–1478. doi:10.1093/molbev/msx094. ISSN 0737-4038. PMC 5435084. PMID 28333212.
- ^ Dennis, Megan Y.; Nuttle, Xander; Sudmant, Peter H.; Antonacci, Francesca; Graves, Tina A.; Nefedov, Mikhail; Rosenfeld, Jill A.; Sajjadian, Saba; Malig, Maika; Kotkiewicz, Holland; Curry, Cynthia J.; Shafer, Susan; Shaffer, Lisa G.; de Jong, Pieter J.; Wilson, Richard K.; Eichler, Evan E (2012-05-11). "Human-specific evolution of novel SRGAP2 genes by incomplete segmental duplication". Cell. 149 (4): 912–922. doi:10.1016/j.cell.2012.03.033. ISSN 0092-8674. PMC 3365555. PMID 22559943.
- ^ Charrier, Cécile; Joshi, Kaumudi; Coutinho-Budd, Jaeda; Kim, Ji-Eun; Lambert, Nelle; de Marchena, Jacqueline; Jin, Wei-Lin; Vanderhaeghen, Pierre; Ghosh, Anirvan; Sassa, Takayuki; Polleux, Franck (2012-05-11). "Inhibition of SRGAP2 function by its human-specific paralogs induces neoteny during spine maturation". Cell. 149 (4): 923–935. doi:10.1016/j.cell.2012.03.034. ISSN 0092-8674. PMC 3357949. PMID 22559944.
- ^ "OMIM Entry - * 606524 - SLIT-ROBO RHO GTPase-ACTIVATING PROTEIN 2; SRGAP2". Online Mendelian Inheritance in Man. (Archive version from 25 November 2020.)